How to Characterize Protein Using Microfluidic Modulation Spectroscopy
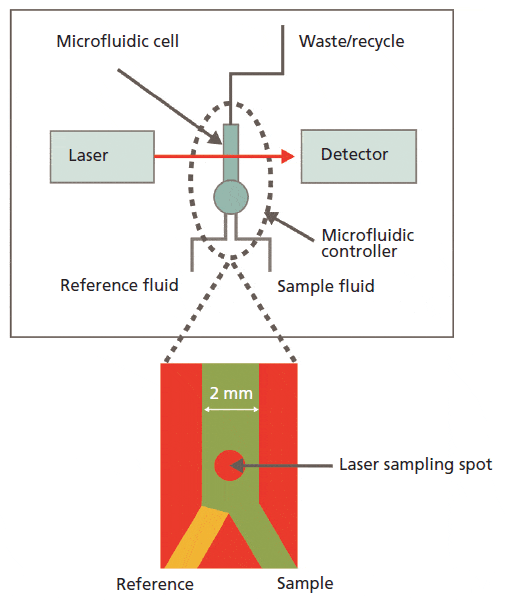
Protein characterization using microfluidic modulation spectroscopy enables the collection of high-quality Circular Dichroism data from monoclonal antibodies (mAbs) at low formulation concentrations. The technique utilizes a tunable quantum cascade laser that is 1000 times more intense than incumbent IR technologies.
CD spectroscopy is a vital tool for understanding how a therapeutic proteins behaves in different conditions and formulations. This includes evaluating aggregation behavior, assessing process-related changes in structure for biosimilar development and optimizing drug formulations.
What is microfluidic modulation spectroscopy?
Proteins are large molecules with complex three-dimensional (3D) structures that directly influence their efficacy and safety as biotherapeutics. The primary structure of proteins is encoded by the amino acid sequences of their peptide backbones with secondary structures formed by interactions between hydrogen bond donors and acceptors in repeating peptide units to form a-helix and b-strand motifs. During formulation, the stability of these higher order structural motifs is critical to the product’s function.
To assess the stability of proteins and their formulations, scientists use a core set of analytical techniques to quantify the colloidal, chemical and conformational stability parameters that define the stability of a biologic drug substance or formulated product. These tools include Fourier-transform infrared spectroscopy (FTIR) and circular dichroism (CD). Both FTIR and CD are well established as characterization methods for protein secondary structures, but they have limitations in their ability to detect lower-order changes in protein chemistry during formulation.
These limits come from a combination of sample dynamic range issues and the difficulty of obtaining accurate concentration quantitation due to their reliance on light-scattering detectors and limited detector linearity. Additionally, the high-powered quantum cascade laser that enables these instruments is susceptible to interference from atmospheric water vapor in the 2000-1300 cm-1 wavelength region, limiting their sensitivity.
Microfluidic Modulation Spectroscopy (MMS) is a new QCL-based automated IR technology that overcomes these limitations and has proven to be a powerful tool for generating high quality, reproducible secondary structure data for monoclonal antibodies during formulation. MMS uses an innovative a-helix and b-strand selective optical configuration to deliver superior performance to traditional spectroscopic techniques in terms of sensitivity, measurement time, and excipient interference. This webinar will demonstrate how MMS generates high-quality data on the secondary structure of mAb-based biotherapeutics and shows that it can be used to monitor key quality attributes during formulation without the need for labeling or dilution.
Applications of microfluidic modulation spectroscopy
Characterizing higher-order protein structures is a critical task within the biotherapeutic development and formulation process. These proteins are designed to be functionally stable, so it is important that they are produced with the expected structure and do not undergo significant structural changes during manufacturing or formulation. In order to detect these changes, a highly sensitive instrument is required. Traditional analytical techniques such as differential scanning calorimetry and size-exclusion high-performance liquid chromatography (HPLC) are used to screen for these types of structural changes; however, they lack the ability to measure detailed secondary structure.
Microfluidic modulation spectroscopy addresses these limitations with high sensitivity, wide dynamic range, and a simplified, automated workflow. RedShiftBio’s AQS3pro platform, powered by microfluidic modulation spectroscopy, is a robust tool for characterizing protein-based biotherapeutics. Using this system, scientists can make five key measurements of protein secondary structure: aggregation, quantitation, stability, similarity, and structure.
Protein aggregation is one of the most common indicators of instability and can cause therapeutic antibodies to be unsuitable for clinical use. Inhibitors of protein aggregation have the potential to significantly reduce the number of failed drug candidates in the pipeline. In addition to its superior sensitivity, microfluidic modulation spectroscopy can measure previously undetectable changes in protein structure, including the formation of intermolecular b-sheets commonly associated with aggregate formation.
The AQS3pro analyzer uses a tunable mid-infrared quantum cascade laser to produce an optical signal 1000 times brighter than the sources used in conventional FTIR spectroscopy, allowing for measurement of samples at lower concentrations and with simpler detectors without the need for nitrogen cooling. Sample and reference buffer streams are automatically introduced into a microfluidic flow cell and modulated in-line with the laser beam for background compensated, drift-free detection. The spectra are measured and processed by the software to generate second derivative polar plots with peak shifts and area differences compared to a standard sample.
Why do we need to characterize protein?
Proteins are large organic molecules that perform a wide range of vital biological functions and act as the major structural elements of cells. They are composed of chains of amino acid residues that assemble into three-dimensional structures and vary in size. The specific sequence of amino acids that makes up a protein’s primary structure determines its chemical properties and nutritional attributes. Proteins fold into complex spatial conformations driven by non-covalent interactions that stabilize via hydrogen bonding, ionic interactions, van der Waals forces and hydrophobic packing. This folding leads to the formation of secondary and tertiary molecular structures. Proteins can also bind to each other forming higher order structures such as dimer, trimer or oligomer.
The larger size, complexity and intrinsic nature of proteins make protein analysis and characterization more difficult than other molecules. Whether purified from natural sources, as a result of the manufacturing process or during drug development, a broad range of impurities and contaminants can impact the final product. These contaminants and processing-related impurities must be accounted for in determining the safety and efficacy of biotherapeutics.
A core set of analytical techniques is available to quantify colloidal, chemical and conformational stability in clinically representative formulations. These include size exclusion chromatography (SEC), capillary electrophoresis and mass spectrometry. However, these tools have well-documented limitations, particularly their inability to distinguish conformational differences with high repeatability.
A new analytical technique, microfluidic modulation spectroscopy (MMS), addresses these challenges by directly probing protein secondary structure. MMS enables direct label free quantitation over a broad concentration range and is more selective and less susceptible to interferences than alternative protein characterization technologies. MMS offers a more efficient and cost effective method to assess the structural integrity of biopharmaceuticals compared to traditional techniques.
Circular dichroism
In the near and far-ultraviolet wavelength ranges, chiral chromophores such as amino acid carbonyls and amides in protein backbones absorb left- and right-circularly polarized light differently. This produces a circular dichroism (CD) signal with wavelengths and magnitudes that can be used to characterize the secondary structural content of proteins. In particular, a-helix, b-strand and unordered structure are easily identified in protein CD spectra, while ligand binding and conformational changes can also be monitored by comparing a wild-type protein to its mutant.
The spectral information contained in a CD spectrum is proportional to the rotational symmetry of a protein, and a number of methods have been developed for analysing and interpreting CD data. A protein’s atomic coordinates can also be used to calculate its CD spectra, providing a means of directly comparing two proteins even when only the X-ray crystallography, cryo-electron microscopy or NMR structure for one is available. This can be very useful in validating homology models, determining the effect of a mutation on a protein’s conformation and monitoring protein-ligand interactions.
A large number of software packages exist for interpreting CD data, but it is important to be aware that different instruments produce slightly different data sets. Therefore, it is necessary to use a common file format that allows data to be readily compared across different instruments and analytical packages. For this purpose, a common spectral file format has been developed called CDToolX13 which can be used with any instrument to generate output results that can be used with other tools for comparisons and secondary structure analysis. The Protein Circular Dichroism Data Bank (PCDDB35) is a freely-accessible repository of both experimental and back-calculated protein CD data that can be used with these tools, as well as with other bioinformatics tools for the study of proteins.
Fourier transform infrared spectroscopy
Fourier transform infrared spectroscopy is an analytical technique used to identify organic, polymeric and some inorganic materials. It scans a sample of a chemical substance and observes the vibrational modes that occur in the molecules. These vibrational modes are either Raman active (scattered due to change in polarizability) or IR active (absorption of light due to a change in dipole moment). Two primary modes of vibration are bending and stretching. These can be distinguished because the vibrational frequencies of these two states differ. Typically, the bending vibrations have lower frequency than the stretching vibrations.
FT-IR spectra can be collected in a variety of ways. The most common method is transmission, where the IR spectrometer shines a beam of light through the sample at a certain angle. The light is then reflected off of the surface of the sample and detected. Reflection IR spectroscopy is also available, but is less commonly used. With this method, the IR spectrometer is focusing on the surface of the sample and the reflections off of it. The spectra that is collected are then measured and recorded by the spectrometer.
These spectra can be used for both identification and quantification. In order to quantify a sample, the spectra are compared to a known reference spectral library. The peaks that match the reference spectrum are then plotted, and the concentration of each component in the sample can be determined.
FT-IR has many applications in protein characterization. For example, it can be used to detect alterations in the structure of a protein. For example, a recent study showed that FT-IR analysis could distinguish between blood samples of leukaemia patients and healthy controls by analyzing a peak that corresponds to C-H bond stretching. This finding was made by comparing the ratios of corrected peaks heights of the IR spectra of ten leukaemia patient samples to those of ten control samples.