Electron Microscope – Its Working Principles and Component Parts
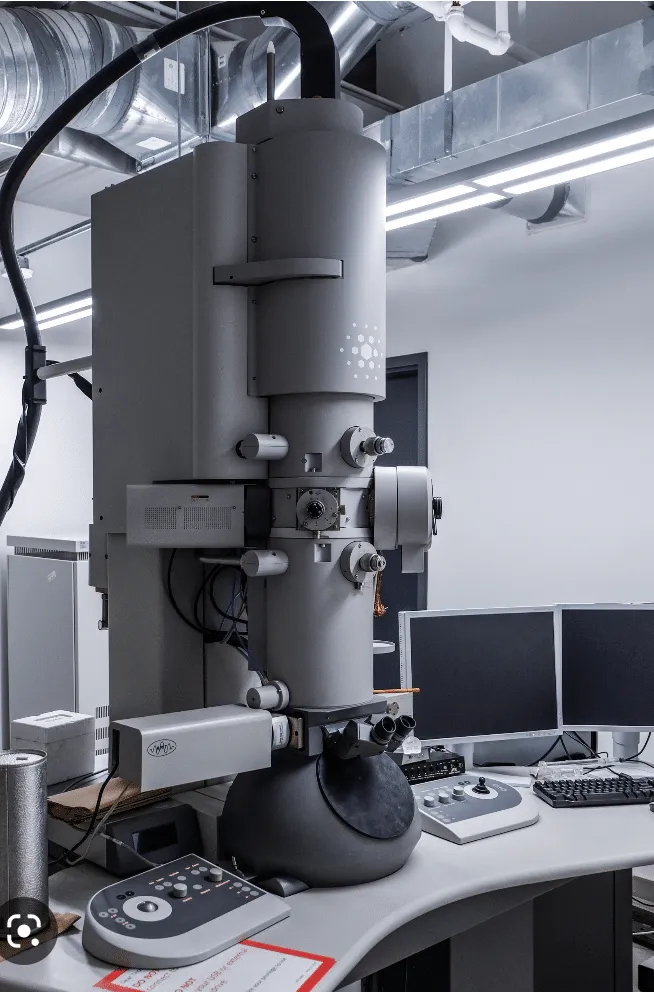
Have you ever wanted a closer look at the world around us? Whether it’s studying microscopic organisms or investigating the structure of materials, an electron microscope is a powerful tool for conducting research. This blog article takes a look at the working principles and components of an electron microscope. By understanding its capabilities and limitations, you can make better use of this instrument for your scientific pursuits. Read on to learn more about how an electron microscope works and what components are necessary for its operation.
How Does an Electron Microscope Work?
An electron microscope is a type of microscope that uses a beam of electrons to create an image of the specimen. This microscopes are used to examine the structure of small objects such as cells, viruses, and bacteria.
An electron microscope uses a beam of electrons to examine the specimen. The electrons interact with the atoms in the specimen, and these interactions produce a signal that is used to create an image of the specimen.
The first electron microscopes were created in the 1930s, and they have since undergone several major improvements. Today, there are two main types of electron microscopes: transmission electron microscopes (TEMs) and scanning electron microscopes (SEMs).
TEMs use a beam of electrons that passes through the specimen to create an image. The electrons interact with the atoms in the specimen, and these interactions produce a signal that is used to create an image of the specimen. SEMs use a beam of electrons that scans across the surface of the specimen to create an image. The interaction between the electrons and atoms in the specimen produces a signal that is used to create an image of the surface of the specimen.
Types of Electron Microscopes
When it comes to electron microscopes, there are two main types:
1. Transmission Electron Microscopes (TEMs) and
2. Scanning Electron Microscopes (SEMs).
Each type of electron microscope has its own unique working principle and set of components.
TEMs work by firing a beam of electrons through a very thin specimen. The electrons that make it through the specimen are then detected on the other side. This allows for the visualization of extremely small details within the specimen.
SEMs work by scanning a focused beam of electrons over the surface of a specimen. The electrons that interact with the atoms on the surface cause them to emit secondary electrons. These secondary electrons are then detected and used to create an image of the surface of the specimen.
The Parts of an Electron Microscope
An electron microscope is an instrument that uses a beam of electrons to create a magnified image of a specimen. The three main parts of an electron microscope are the electron gun, which produces the electron beam, the lenses, which focus the beam, and the stage, on which the specimen is placed.
The electron gun is the part of the microscope that produces the electron beam. It is made up of two parts: the cathode, which emits electrons, and the anode, which accelerates them. The cathode is usually made of tungsten or another material that can emit electrons when heated. The anode is made of a metal such as copper or molybdenum and has a hole in it through which the electron beam passes.
The lenses are what focus the electron beam onto the specimen. There are two types of lenses: electrostatic lenses and magnetic lenses. Electrostatic lenses use electric fields to focus the beam, while magnetic lenses use magnetic fields. Magnetic lenses are used more often because they can produce a higher resolution image.
The stage is where the specimen is placed during imaging. It is important that the stage be able to hold the specimen securely while it is being bombarded by electrons. The stage also has a heating element so that specimens can be kept at a desired temperature during imaging.
Advantages and Disadvantages of Using an Electron Microscope
An electron microscope is a powerful tool that can provide researchers with extremely detailed images of very small objects. However, there are some disadvantages to using this type of microscope. One potential disadvantage is that the images produced by an electron microscope can be difficult to interpret. This is because the level of detail can be so high that it can be hard to distinguish one object from another. Additionally, electron microscopes are expensive, and they require specialized training to use effectively.
The electron microscope is an incredibly powerful tool used in many scientific and medical applications. Its ability to magnify objects at a very high level allows us to view details that would be impossible with traditional optical microscopes. This article has provided a brief overview of the working principles and components of the electron microscope, as well as some of its common uses. With further research, you can learn more about this sophisticated instrument and what it has to offer.
Electron Microscope and Its Important Component Parts
Electron microscopes (EM) are similar to their optical counterparts, but use a focused beam of electrons instead of light to image specimens. Electrons are produced from an electron gun and scanned over the sample surface by electromagnetic coils. Backscattered electrons are detected by a detector and relayed to a screen for display.
Electron Gun
The electron gun (shown in Figure 1(A)) is the source of the electron beam for the SEM. It consists of a heated V-shaped tungsten filament or, in high performance instruments, a sharply pointed rod of lanthanum hexaboride. The filament is surrounded by a control grid, also called the Wehnelt cylinder, and a central aperture arranged on the axis of the column. The cathode and the control grid are held at a negative potential equal to the desired accelerating voltage. The central aperture of the electron gun serves as an electron beam injector, directing the injected electrons close to the target atoms. This design reduces back accelerated electrons that may hit the cathode, and it is very effective at mitigating magnetic field effects on the gun.
The geometry of the cathode and the grid is very important to the performance of an electron microscope. Various gun metrics, such as the emission current, the injection current, and the efficiency in trace space are strongly dependent on this geometry. In addition, the cathode geometry can influence sensitivity of the electron gun to in-line magnetic fields.
Electrons emitted at the cathode are accelerated by the negative voltage of the control grid and pass through the central aperture to strike the target atoms. Inelastic scattering causes four signals: secondary electrons, characteristic x-rays, bremsstrahlung radiation, and cathodoluminescence radiation. The amount of backscattered electrons is a function of the atomic number of the sample. Increasing the energy of the electron beam results in more backscattered electrons.
Between the electron gun and the specimen stage, there is a set of condenser lenses used to manipulate the intensity and angular aperture of the beam. This lens system is especially important to ensure that the electron beam does not cause specimen charging. Ideally, the beam is focused into a very fine spot at the surface of the specimen.
To do this, the last manipulation of the beam takes place in a final lens, which is at the heart of the SEM. This lens contains raster coils that raster or scan the focused beam over the specimen. This scanning, in turn, produces the image on the screen.
Electron Lenses
Electron microscopes operate much like their optical counterparts except they use a stream of electrons instead of photons to image specimens and gain information about their structure and composition. A high voltage electric current forms a stream of electrons from an electron gun and is accelerated in a vacuum toward the sample using metal apertures and magnetic lenses. As the electrons approach the specimen they interact with the atoms of the specimen and the lens itself, producing various effects that are detected and transformed into an image.
The magnification of an electron microscope is determined by the size of the specimen’s spot on the detector. The smaller the spot, the higher the magnification. The first condenser lens or C1 determines the spot size by reducing the diameter of the primary beam that is emitted from the electron gun. The second condenser, or C2, reduces the spot size by excluding off-axis electrons and further narrowing the primary beam. This is called demagnification.
A magnetic lens in an electron microscope consists of a solenoid of copper wire. The shape of the pole piece (the part of the magnetic circuit with a hole and gap) determines how the lens functions. As the electrons pass through the lens, they are bended toward the lens axis and form a spot on the detector that corresponds to the position of the sample in the x-y plane.
All electromagnetic lenses suffer from some aberrations including spherical aberration, distortion, coma, astigmatism and chromatic aberration. These aberrations can be minimized by optimizing the lens design and by controlling the operating conditions of the instrument.
For SEMs, the location of the primary beam is scanned across the surface of the specimen by currents in scan coils situated within the final lens. This produces a variety of signals such as secondary electrons, backscattered electrons and characteristic X-rays. The signals are detected by appropriate detectors and relayed to an electronic console, from which the image is displayed. It is also possible to overlay images from light and electron microscopy of the same area of a specimen for correlative analysis.
Condenser Lens
When you turn the knob that moves the condenser closer or further from the specimen stage on a microscope, it changes the amount of light the lens lets through. This is called changing the condenser aperture. It also adjusts the size of the light cone that illuminates the sample. Similarly, the lever that controls the opening or closing of the aperture diaphragm that’s built into the condenser (or just below it) is used to change the intensity and focus of illumination on the sample.
In this way, the condenser defines the size of the electron beam before it’s converged by the objective front lens and hits the sample. It’s important that the condenser be adjusted correctly to provide a light cone that matches the numerical aperture of the highest power objective on your microscope.
There are many different types of electromagnetic lenses that can be used in a microscope. The simplest is an Abbe condenser, named after Ernst Abbe who invented it in the 1800s. These are typically found on less expensive microscopes. They don’t correct for spherical or chromatic aberrations but are capable of having a relatively large numerical aperture setting such as 1.40.
Other condensers are designed to achieve higher resolution by correcting these aberrations. For example, an aplanatic-achromatic condenser has eight internal lens elements cemented together as two doublets and four singles and has an aperture that can be adjusted to match the numerical aperture of the objective lens.
Once the condenser and the objective lenses have converged a tight beam of electrons onto the sample, the image can be analyzed by various imaging and analytical detectors. The images produced can reveal a wealth of information such as backscattered electrons (to indicate composition), secondary electrons to show surface topography, characteristic x-rays to determine material properties, and more.
The SEM can also be used to directly write fine nanopatterns, capture forensic evidence, inspect metallography, analyze biological samples, and pinpoint quality problems. Let JEOL help you select the best instrument for your applications. Call or email us for applications support and expert training.
Detectors
Detectors are the main element of the microscope that capture images and allow microanalysis. In the modern SEM this includes both electron emission scanning detectors (ESD) and energy dispersive X-ray spectroscopy detectors (EDS).
Electrons from the gun travel through a condenser lens and then the sample before passing through a specimen mirror. This defines the size of the focused electron spot that interacts with the material. It is this interaction that creates the image. Electrons that are not scattered by the specimen are reflected back into the microscope column. This is what makes the SEM so effective at generating 3D images of lice, flies and snowflakes.
The current generation of pixelated detectors use silicon, which is highly effective at converting the high-energy electrons’ kinetic energy into electron-hole pairs. This leads to an electronic signal that is converted to a digital picture via photomultiplier tubes or other electronics. This picture can then be displayed on a monitor or captured by a CCD camera for viewing.
As the technology has advanced, the ability of SEMs to record higher-resolution images in a shorter time has increased. This has allowed the instrument to be used in a wide range of industrial, commercial and research applications, from cutting edge manufacturing processes to forensic science.
One of the most important advancements has been the implementation of electron pulse counting in the microscope’s detectors. This hardware discretises the electron signal in real-time and synchronises it with the scanner control system without compromising existing analog or spectroscopic signals. This enables the acquisition of quantitative measurements rather than the traditional digitisation values and removes Gaussian noise from the data. It also allows image intensity units to be derived from the probability of an incoming electron scattering in the detector.
Another major advance is the emergence of field-emission gun (FEG) sources. This is a thin wire of tungsten with a very sharp tip, less than 100 nm, that generates the electron beam through electron tunnelling, avoiding the need for an electric field at the sample surface. The resulting electron beam is very bright and highly coherent, allowing high resolutions to be obtained.